GXF Development Guide¶
This section covers upon the guide that helps in understanding the basic building blocks of GXF pipeline. We will go through two workflows - Application Development Guide and Extensions Development Guide
Application Development Guide¶
This section explains about the core concepts of GXF required for application development. One of the key components in the application development is the Codelets and it is important to understand its lifecycle.
LifeCycle of a Codelet¶
The life cycle of a codelet is governed by the below five stages
Initialize: Used for light-weight initialization. Called directly when the codelet is created for the first time. Called exactly once over the lifetime of a codelet.
Deinitialize: Used for light-weight deinitialization. Called directly before the codelet is destroyed. Called exactly once over the lifetime of a codelet.
Start: Used for heavy-weight initialization to allocate resources which are necessary for execution. Can be called multiple times over the lifetime of the codelet according to order defined by the lifecycle.
Stop: Used for heavy-weight deinitialization to deallocate all resources which were allocated by the start function. Can be called multiple times over the lifetime of the codelet according to order defined by the lifecycle.
Tick: Called when the codelet is triggered. Triggering can happen when data arrives or based on a time trigger. Normally called many times over the lifetime of a codelet.
Codelet component is used to define a custom codelet that will accomplish the desired work. Below example describes on how to implement a custom codelet. For now please ignore :ref: registerInterface which will covered in the later sections.
1 2 3 4 5 6 7 8 9 10 11 12 13 14 15 16 17 18 19 20 class Test : public Codelet { public: gxf_result_t start() override { return GXF_SUCCESS; } gxf_result_t tick() override { // Do Something ... return GXF_SUCCESS; } gxf_result_t stop() override { return GXF_SUCCESS; } gxf_result_t registerInterface(Registrar* registrar) override { Expected<void> result; result &= registrar->parameter(some_parameter_, "Some Parameter"); return ToResultCode(result); } Parameter<Handle<Receiver>> some_parameter_; };
- Below example shows on how to create a simple custom codelet that prints “Hello World”
1 2 3 4 5 6 7 8 9 10 11 12 13 14 15 16 17 18 19 20
class HelloWorld : public Codelet { public: gxf_result_t start() override { return GXF_SUCCESS; } gxf_result_t tick() override { GXF_LOG_INFO("Hello World from GXF"); return GXF_SUCCESS; } gxf_result_t stop() override { return GXF_SUCCESS; } gxf_result_t registerInterface(Registrar* registrar) override { Expected<void> result; result &= registrar->parameter(some_parameter_, "Some Parameter"); return ToResultCode(result); } Parameter<Handle<Receiver>> some_parameter_; };
- Below example explores on how to use the initialize/deinitialize and start/stop stages
1 2 3 4 5 6 7 8 9 10 11 12 13 14 15 16 17 18 19 20 21 22 23 24 25 26 27 28 29 30 31 32 33 34 35 36 37 38 39 40 41 42 43 44 45 46 47 48
class File : public Codelet { public: gxf_result_t initialize() { access_file_ = true; return GXF_SUCCESS; } gxf_result_t start() override { if (access_file_) { file_ = fopen("Some File Name", "Read or Write Mode"); if (file_ == nullptr) { GXF_LOG_ERROR("%s", strerror(errno)); return Unexpected{GXF_FAILURE}; } } return GXF_SUCCESS; } gxf_result_t tick() override { // Do some file operation return GXF_SUCCESS; } gxf_result_t stop() override { const int result = fclose(file_); if (result != 0) { GXF_LOG_ERROR("%s", strerror(errno)); return Unexpected{GXF_FAILURE}; } file_ = nullptr; return GXF_SUCCESS; } gxf_result_t deinitialize() override { access_file_ = false; return GXF_SUCCESS; } gxf_result_t registerInterface(Registrar* registrar) override { Expected<void> result; result &= registrar->parameter(some_parameter_, "Some Parameter"); // Not used here return ToResultCode(result); } Parameter<Handle<Receiver>> some_parameter_; bool access_file_{false}; std::FILE* file_; };
The GXF Scheduler¶
The execution of entities in a graph is governed by the scheduler and the scheduling terms associated with every entity. A scheduler is a component responsible for orchestrating the execution of all the entities defined in a graph. A scheduler typically keeps track of the graph entities and their current execution states and passes them on to a nvidia::gxf::EntityExecutor component when ready for execution. The following diagram depicts the flow for an entity execution.
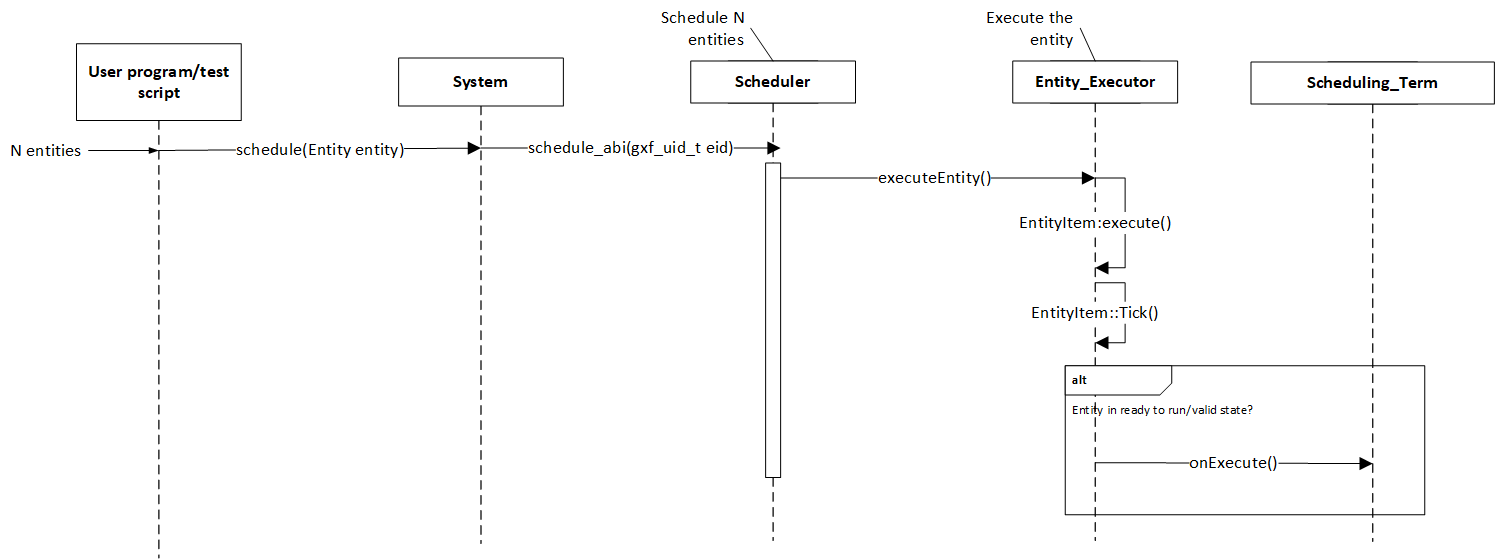
Figure: Entity execution sequence
As shown in the sequence diagram, the schedulers begin executing the graph entities via the nvidia::gxf::System::runAsync_abi() interface and continue this process until it meets the certain ending criteria. A single entity can have multiple codelets. These codelets are executed in the same order in which they were defined in the entity. A failure in execution of any single codelet stops the execution of all the entities. Entities are naturally unscheduled from execution when any one of their scheduling term reaches NEVER state.
Scheduling terms are components used to define the execution readiness of an entity. An entity can have multiple scheduling terms associated with it and each scheduling term represents the state of an entity using SchedulingCondition.
The table below shows various states of nvidia::gxf::SchedulingConditionType described using nvidia::gxf::SchedulingCondition.
SchedulingConditionType |
Description |
---|---|
NEVER |
Entity will never execute again |
READY |
Entity is ready for execution |
WAIT |
Entity may execute in the future |
WAIT_TIME |
Entity will be ready for execution after specified duration |
WAIT_EVENT |
Entity is waiting on an asynchronous event with unknown time interval |
Schedulers define deadlock as a condition when there are no entities which are in READY, WAIT_TIME or WAIT_EVENT state which guarantee execution at a future point in time. This implies all the entities are in WAIT state for which the scheduler does not know if they ever will reach the READY state in the future. The scheduler can be configured to stop when it reaches such a state using the stop_on_deadlock parameter, else the entities are polled to check if any of them have reached READY state. max_duration config parameter can be used to stop execution of all entities regardless of their state after a specified amount of time has elapsed.
There are three types of schedulers currently supported by GXF
Greedy Scheduler
Multithread Scheduler
Epoch Scheduler
Greedy Scheduler¶
This is a basic single threaded scheduler which tests scheduling term greedily. It is great for simple use cases and predictable execution but may incur a large overhead of scheduling term execution, making it unsuitable for large applications. The scheduler requires a clock to keep track of time. Based on the choice of clock the scheduler will execute differently. If a Realtime clock is used the scheduler will execute in real-time. This means pausing execution - sleeping the thread, until periodic scheduling terms are due again. If a ManualClock is used scheduling will happen “time-compressed”. This means flow of time is altered to execute codelets in immediate succession.
The GreedyScheduler maintains a running count of entities which are in READY, WAIT_TIME and WAIT_EVENT states. The following activity diagram depicts the gist of the decision making for scheduling an entity by the greedy scheduler -
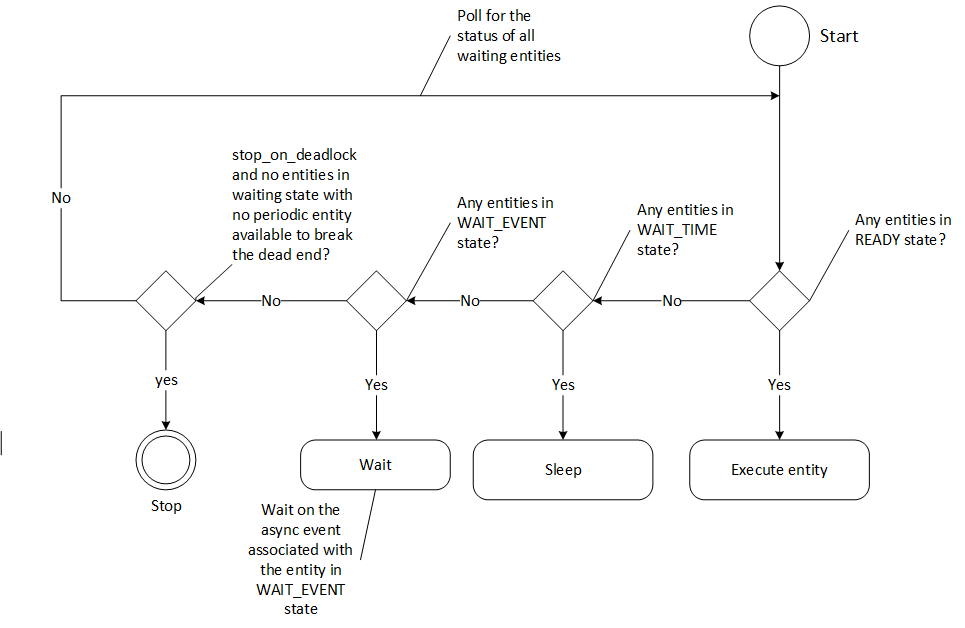
Figure: Greedy Scheduler Activity Diagram
Greedy Scheduler Configuration¶
The greedy scheduler takes in the following parameters from the configuration file
Parameter name |
Description |
---|---|
clock |
The clock used by the scheduler to define the flow of time. Typical choices are RealtimeClock or ManualClock |
max_duration_ms |
The maximum duration for which the scheduler will execute (in ms). If not specified, the scheduler will run until all work is done. If periodic terms are present this means the application will run indefinitely |
stop_on_deadlock |
If stop_on_deadlock is disabled, the GreedyScheduler constantly polls for the status of all the waiting entities to check if any of them are ready for execution. |
Example usage - The following code snippet configures a Greedy scheduler with a ManualClock option specified.
name: scheduler
components:
- type: nvidia::gxf::GreedyScheduler
parameters:
max_duration_ms: 3000
clock: misc/clock
stop_on_deadlock: true
---
name: misc
components:
- name: clock
type: nvidia::gxf::ManualClock
Multithread Scheduler¶
The MultiThread scheduler is more suitable for large applications with complex execution patterns. The scheduler consists of a dispatcher thread which checks the status of an entity and dispatches it to a thread pool of worker threads responsible for executing them. Worker threads enqueue the entity back on to the dispatch queue upon completion of execution. The number of worker threads can be configured using worker_thread_number parameter. The MultiThread scheduler also manages a dedicated queue and thread to handle asynchronous events. The following activity diagram demonstrates the gist of the multithread scheduler implementation.
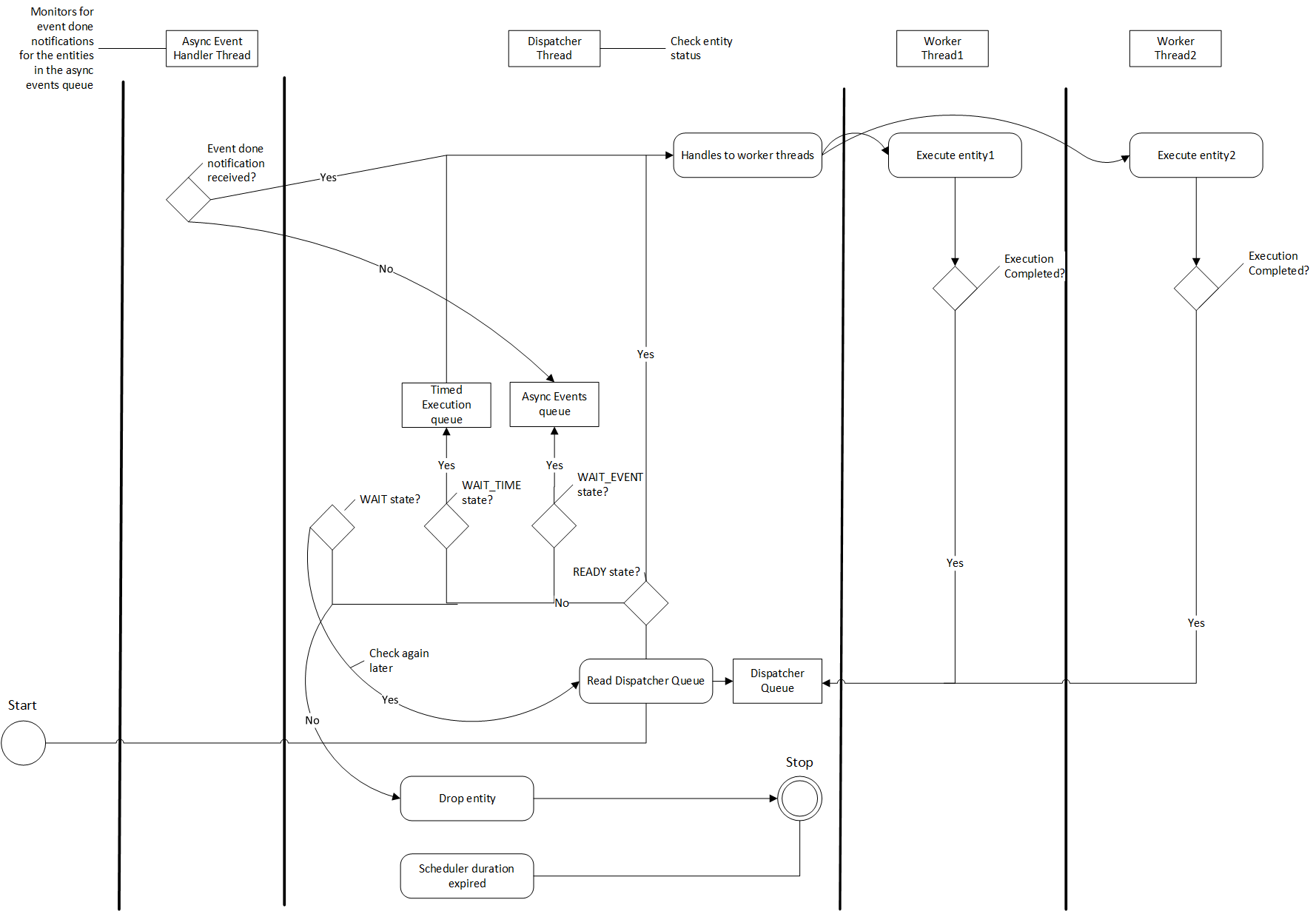
Figure: MultiThread Scheduler Activity Diagram
As depicted in the diagram, when an entity reaches WAIT_EVENT state, it’s moved to a queue where they wait to receive event done notification. The asynchronous event handler thread is responsible for moving entities to the dispatcher upon receiving event done notification. The dispatcher thread also maintains a running count of the number of entities in READY, WAIT_EVENT and WAIT_TIME states and uses these statistics to check if the scheduler has reached a deadlock. The scheduler also needs a clock component to keep track of time and it is configured using the clock parameter.
MultiThread scheduler is more resource efficient compared to the Greedy Scheduler and does not incur any additional overhead for constantly polling the states of scheduling terms. The check_recession_period_ms parameter can be used to configure the time interval the scheduler must wait to poll the state of entities which are in WAIT state.
The multithread scheduler takes in the following parameters from the configuration file
Parameter name |
Description |
---|---|
clock |
The clock used by the scheduler to define the flow of time. Typical choices are RealtimeClock or ManualClock. |
max_duration_ms |
The maximum duration for which the scheduler will execute (in ms). If not specified, the scheduler will run until all work is done. If periodic terms are present this means the application will run indefinitely. |
check_recess_period_ms |
Duration to sleep before checking the condition of an entity again [ms]. This is the maximum duration for which the scheduler would wait when an entity is not yet ready to run. |
stop_on_deadlock |
If enabled the scheduler will stop when all entities are in a waiting state, but no periodic entity exists to break the dead end. Should be disabled when scheduling conditions can be changed by external actors, for example by clearing queues manually. |
worker_thread_number |
Number of threads. |
Example usage - The following code snippet configures a Multithread scheduler with the number of worked threads and max duration specified -
name: scheduler
components:
- type: nvidia::gxf::MultiThreadScheduler
parameters:
max_duration_ms: 5000
clock: misc/clock
worker_thread_number: 5
check_recession_period_ms: 3
stop_on_deadlock: false
---
name: misc
components:
- name: clock
type: nvidia::gxf::RealtimeClock
Epoch Scheduler¶
The Epoch scheduler is used for running loads in externally managed threads. Each run is called an Epoch. The scheduler goes over all entities that are known to be active and executes them one by one. If the epoch budget is provided (in ms), it would keep running all codelets until the budget is consumed or no codelet is ready. It might run over budget since it guarantees to cover all codelets in epoch. In case the budget is not provided, it would go over all the codelets once and execute them only once.
The epoch scheduler takes in the following parameters from the configuration file -
Parameter name |
Description |
---|---|
clock |
The clock used by the scheduler to define the flow of time. Typical choice is a RealtimeClock. |
Example usage - The following code snippet configures an Epoch scheduler -
name: scheduler
components:
- name: clock
type: nvidia::gxf::RealtimeClock
- name: epoch
type: nvidia::gxf::EpochScheduler
parameters:
clock: clock
Note that the epoch scheduler is intended to run from an external thread. The runEpoch(float budget_ms);
can be used to set the budget_ms and run the scheduler from the external thread. If the specified budget is not positive, all the nodes are executed once.
SchedulingTerms¶
A SchedulingTerm defines a specific condition that is used by an entity to let the scheduler know when it’s ready for execution. There are various scheduling terms currently supported by GXF. If multiple scheduling terms are present in an entity they all have to be true for an entity to execute.
PeriodicSchedulingTerm¶
An entity associated with nvidia::gxf::PeriodicSchedulingTerm is ready for execution after periodic time intervals specified using its recess_period parameter. The PeriodicSchedulingTerm can either be in READY or WAIT_TIME state.
Example usage -
- name: scheduling_term
type: nvidia::gxf::PeriodicSchedulingTerm
parameters:
recess_period: 50000000
CountSchedulingTerm¶
An entity associated with nvidia::gxf::CountSchedulingTerm is executed for a specific number of times specified using its count parameter. The CountSchedulingTerm can either be in READY or NEVER state. The scheduling term reaches the NEVER state when the entity has been executed count number of times.
Example usage -
- name: scheduling_term
type: nvidia::gxf::CountSchedulingTerm
parameters:
count: 42
MessageAvailableSchedulingTerm¶
An entity associated with nvidia::gxf::MessageAvailableSchedulingTerm
is executed when the associated receiver queue has at least a certain number of elements.
The receiver is specified using the receiver
parameter of the scheduling term. The minimum number of messages that permits the execution of the entity is specified by min_size
. An optional parameter for this scheduling term is front_stage_max_size
, the maximum front stage message count. If this parameter is set, the scheduling term will only allow execution if the number of messages in the queue does not exceed this count. It can be used for codelets which do not consume all messages from the queue.
In the example shown below, the minimum size of the queue is configured to be 4. So, the entity will not be executed till there are at least 4 messages in the queue.
- type: nvidia::gxf::MessageAvailableSchedulingTerm
parameters:
receiver: tensors
min_size: 4
MultiMessageAvailableSchedulingTerm¶
An entity associated with nvidia::gxf::MultiMessageAvailableSchedulingTerm
is executed when a list of provided input receivers combined have at least a given number of messages. The receivers
parameter is used to specify a list of the input channels/receivers. The minimum number of messages needed to permit the entity execution is set by min_size
parameter.
Consider the example shown below. The associated entity will be executed when the number of messages combined for all the three receviers is at least the min_size, i.e. 5.
- name: input_1
type: nvidia::gxf::test::MockReceiver
parameters:
max_capacity: 10
- name: input_2
type: nvidia::gxf::test::MockReceiver
parameters:
max_capacity: 10
- name: input_3
type: nvidia::gxf::test::MockReceiver
parameters:
max_capacity: 10
- type: nvidia::gxf::MultiMessageAvailableSchedulingTerm
parameters:
receivers: [input_1, input_2, input_3]
min_size: 5
BooleanSchedulingTerm¶
An entity associated with nvidia::gxf::BooleanSchedulingTerm
is executed when its internal state is set to tick. The parameter enable_tick
is used to control the entity execution. The scheduling term also has two APIs enable_tick()
and disable_tick()
to toggle its internal state. The entity execution can be controlled by calling these APIs. If enable_tick
is set to false, the entity is not executed (Scheduling condition is set to NEVER
). If enable_tick
is set to true, the entity will be executed (Scheduling condition is set to READY
). Entities can toggle the state of the scheduling term by maintaining a handle to it.
Example usage -
- type: nvidia::gxf::BooleanSchedulingTerm
parameters:
enable_tick: true
AsynchronousSchedulingTerm¶
AsynchronousSchedulingTerm is primarily associated with entities which are working with asynchronous events happening outside of their regular execution performed by the scheduler. Since these events are non-periodic in nature, AsynchronousSchedulingTerm prevents the scheduler from polling the entity for its status regularly and reduces CPU utilization. AsynchronousSchedulingTerm can either be in READY, WAIT, WAIT_EVENT or NEVER states based on asynchronous event it’s waiting on.
The state of an asynchronous event is described using nvidia::gxf::AsynchronousEventState and is updated using the setEventState API.
AsynchronousEventState |
Description |
---|---|
READY |
Init state, first tick is pending |
WAIT |
Request to async service yet to be sent, nothing to do but wait |
EVENT_WAITING |
Request sent to an async service, pending event done notification |
EVENT_DONE |
Event done notification received, entity ready to be ticked |
EVENT_NEVER |
Entity does not want to be ticked again, end of execution |
Entities associated with this scheduling term most likely have an asynchronous thread which can update the state of the scheduling term outside of it’s regular execution cycle performed by the gxf scheduler. When the scheduling term is in WAIT state, the scheduler regularly polls for the state of the entity. When the scheduling term is in EVENT_WAITING state, schedulers will not check the status of the entity again until they receive an event notification which can be triggered using the GxfEntityEventNotify api. Setting the state of the scheduling term to EVENT_DONE automatically sends this notification to the scheduler. Entities can use the EVENT_NEVER state to indicate the end of its execution cycle.
Example usage -
- name: async_scheduling_term
type: nvidia::gxf::AsynchronousSchedulingTerm
DownstreamReceptiveSchedulingTerm¶
This scheduling term specifies that an entity shall be executed if the receiver for a given transmitter can accept new messages.
Example usage -
- name: downstream_st
type: nvidia::gxf::DownstreamReceptiveSchedulingTerm
parameters:
transmitter: output
min_size: 1
TargetTimeSchedulingTerm¶
This scheduling term permits execution at a user-specified timestamp. The timestamp is specified on the clock provided.
Example usage -
- name: target_st
type: nvidia::gxf::TargetTimeSchedulingTerm
parameters:
clock: clock/manual_clock
ExpiringMessageAvailableSchedulingTerm¶
This scheduling waits for a specified number of messages in the receiver. The entity is executed when the first message received in the queue is expiring or when there are enough messages in the queue. The receiver
parameter is used to set the receiver to watch on. The parameters max_batch_size
and max_delay_ns
dictate the maximum number of messages to be batched together and the maximum delay from first message to wait before executing the entity respectively.
In the example shown below, the associated entity will be executed when the number of messages in the queue is greated than max_batch_size
, i.e 5, or when the delay from the first message to current time is greater than max_delay_ns
, i.e 10000000.
- name: target_st
type: nvidia::gxf::ExpiringMessageAvailableSchedulingTerm
parameters:
receiver: signal
max_batch_size: 5
max_delay_ns: 10000000
clock: misc/clock
AND Combined¶
An entity can be associated with multiple scheduling terms which define it’s execution behavior. Scheduling terms are AND combined to describe the current state of an entity. For an entity to be executed by the scheduler, all the scheduling terms must be in READY state and conversely, the entity is unscheduled from execution whenever any one of the scheduling term reaches NEVER state. The priority of various states during AND combine follows the order NEVER, WAIT_EVENT, WAIT, WAIT_TIME, and READY.
Example usage -
components:
- name: integers
type: nvidia::gxf::DoubleBufferTransmitter
- name: fibonacci
type: nvidia::gxf::DoubleBufferTransmitter
- type: nvidia::gxf::CountSchedulingTerm
parameters:
count: 100
- type: nvidia::gxf::DownstreamReceptiveSchedulingTerm
parameters:
transmitter: integers
min_size: 1
BTSchedulingTerm¶
A BT (Behavior Tree) scheduling term is used to schedule a behavior tree entity itself and its child entities (if any) in a Behavior tree.
Example usage -
name: root
components:
- name: root_controller
type: nvidia::gxf::EntityCountFailureRepeatController
parameters:
max_repeat_count: 0
- name: root_st
type: nvidia::gxf::BTSchedulingTerm
parameters:
is_root: true
- name: root_codelet
type: nvidia::gxf::SequenceBehavior
parameters:
children: [ child1/child1_st ]
s_term: root_st
controller: root_controller
Messages¶
In the GXF graph, many a times Codelets may have to communicate with each other to accomplish the defined pipeline. Messages are the form of communication by codelets with each other. Transmitter is used for transmitting the message and Receiver is used for receiving the message. Messages are transmitted or received at tick() of codelet.
Transmitter¶
All the messages from the transmitter are sent as an entity. The transmitter after encapsulating the
message will give a publish()
call which will send the message to target recipient.
Receiver¶
All the messages from the transmitter are received as an entity. The receiver on getting a tick()
call will give a receive()
call that helps in receiving the message.
Below is an example of transmitter and receiver
Transmitter Example¶
1 2 3 4 5 6 7 8 9 10 11 12 13 14 15 | gxf_result_t PingTx::tick() {
Expected<Entity> message = Entity::New(context());
if (!message) {
GXF_LOG_ERROR("Failure creating message entity.");
return message.error();
}
Expected<Entity> maybe_host_msg = Entity::New(context());
auto int_value = maybe_host_msg.value().add<int32_t>("Integer");
auto value = int_value.value();
*value = 9999;
auto result = signal_->publish(maybe_host_msg.value());
GXF_LOG_INFO("Message Sent: int_value = %d", *value);
return ToResultCode(message);
}
|
Receiver Example¶
1 2 3 4 5 6 7 8 9 10 11 | gxf_result_t PingRx::tick() {
auto message = signal_->receive();
if (!message || message.value().is_null()) {
return GXF_CONTRACT_MESSAGE_NOT_AVAILABLE;
}
auto value = message.value();
auto rx_value = message->findAll<int32_t>();
GXF_LOG_INFO("Message Received: rx_value = %d", *(rx_value->at(0).value()));
return GXF_SUCCESS;
}
|
Memory Management¶
GXF provides a way for allocation and deallocation of memory that would be required by the codelets. There are various types of memory allocation that are provided as mentioned below.
System Memory Allocates specified bytes of system memory which typically would make use of underlying OS calls for allocation of requested memory.
Host Memory Allocates specified bytes of host memory that is page-locked and accessible to the device. Allocating excessive amounts of memory with cudaMallocHost() may degrade system performance, since it reduces the amount of memory available to the system for paging. This memory is best used sparingly to allocate staging areas for data exchange between host and device.
Device Memory Allocates specified bytes of device memory.
GXF provides a component called nvidia::gxf::BlockMemoryPool
which is used tp allocate memory
in multiple/single blocks of same size which can be specified as parameters.
Below example specifies on how to allocate host memory
1 2 3 4 5 6 | - name: host_memory_pool
type: nvidia::gxf::BlockMemoryPool
parameters:
storage_type: 0 # host memory
block_size: 1024
num_blocks: 5
|
Below example specifies on how to allocate device memory
1 2 3 4 5 6 | - name: cuda_device_memory_pool
type: nvidia::gxf::BlockMemoryPool
parameters:
storage_type: 1 # device memory
block_size: 1024
num_blocks: 5
|
Below example specifies on how to allocate system memory
1 2 3 4 5 6 | - name: system_memory_pool
type: nvidia::gxf::BlockMemoryPool
parameters:
storage_type: 2 # system memory
block_size: 1024
num_blocks: 5
|
Below example specifies on how to use allocator with codelets
1 2 3 4 5 6 7 8 9 10 11 12 13 14 15 16 17 18 19 | - name: host_memory_pool
type: nvidia::gxf::BlockMemoryPool
parameters:
storage_type: 0 # host memory
block_size: 1024
num_blocks: 5
- name: cuda_device_memory_pool
type: nvidia::gxf::BlockMemoryPool
parameters:
storage_type: 1 # device memory
block_size: 1024
num_blocks: 5
- name: generator
type: nvidia::gxf::test::cuda::StreamTensorGenerator
parameters:
cuda_tensor_pool: cuda_device_memory_pool
host_tensor_pool: host_memory_pool
|
Extensions Development Guide¶
The composer can be used to create an application using GXF standard or public extensions. However, quite often, a custom codelet may also be required to be developed based on specific needs.
Graph Composer package provides a useful tool to help create new extensions and components.
The extension-dev project located at /opt/nvidia/graph-composer/extension-dev/
helps create the
boiler plate code required to create custom extensions. Check LICENSE.txt for the corresponding licenses.
Install dependencies needed for extension development
$ /opt/nvidia/graph-composer/extension-dev/install_dependencies.sh
Sample GXF Extension¶
A script has been provided to generate a sample extension with a codelet and a graph to test the extension within an application. The generated extension contains a single codelet component that logs a message in the start() and tick() methods once and the graph finishes execution. To use the script, run the following commands.
Generate sample extension called test with a codelet named HelloWorld
$ python3 /opt/nvidia/graph-composer/extension-dev/generate_codelet.py \
--output_dir /tmp/ --extn_name test --codelet_name HelloWorld
$ cd /tmp/test
Within the target directory, the following files are autogenerated: codelet (component), extension and an application. The BUILD files needed to generate the extension libraries using bazel build system are also generated.
Header file - hello_world.hpp
1 2 3 4 5 6 7 8 9 10 11 12 13 14 15 16 17 | #pragma once
#include "gxf/std/codelet.hpp"
namespace sample {
namespace test {
// Logs a message in start() and tick()
class HelloWorld : public nvidia::gxf::Codelet {
public:
gxf_result_t start() override;
gxf_result_t tick() override;
gxf_result_t stop() override { return GXF_SUCCESS; }
};
} // namespace test
} // namespace sample
|
cpp file - hello_world.cpp
1 2 3 4 5 6 7 8 9 10 11 12 13 14 15 16 17 18 | #include "HelloWorld.hpp" // NOLINT
namespace sample {
namespace test {
gxf_result_t HelloWorld::start() {
GXF_LOG_INFO("HelloWorld::start");
return GXF_SUCCESS;
}
gxf_result_t HelloWorld::tick() {
GXF_LOG_INFO("HelloWorld::tick");
return GXF_SUCCESS;
}
} // namespace test
} // namespace sample
|
extension cpp file - test.cpp
1 2 3 4 5 6 7 8 9 10 | #include "HelloWorld.hpp"
#include "gxf/std/extension_factory_helper.hpp"
GXF_EXT_FACTORY_BEGIN()
GXF_EXT_FACTORY_SET_INFO(0xd8629d822909316d, 0xa9ee7410c8c1a7b6, "test",
"A Dummy Example", "", "1.0.0", "NVIDIA");
GXF_EXT_FACTORY_ADD(0xd39d70014cab3ecf, 0xb397c9d200cf9e8d, sample::test::HelloWorld,
nvidia::gxf::Codelet, "Dummy example source codelet.");
GXF_EXT_FACTORY_END()
|
Build the extension for both x86 (default) and the aarch64 (jetson) platforms. The tool also generates a workspace file which creates a bazel workspace to compile and execute the extension.
`
$ cd <extension_path>
$ bazel build ...
$ bazel build ... --config=jetson # For building jetson(aarch64) variant
`
The tool also generates a sample application which executes the HelloWorld codelet 10 times and exits.
application yaml file - apps/app.yaml
%YAML 1.2
---
dependencies:
- extension: test
uuid: d8629d82-2909-316d-a9ee-7410c8c1a7b6
version: 1.0.0
---
name: source
components:
- name: signal
type: sample::test::HelloWorld
- type: nvidia::gxf::CountSchedulingTerm
parameters:
count: 10
---
components:
- type: nvidia::gxf::GreedyScheduler
parameters:
realtime: false
max_duration_ms: 1000000
Entities are defined in the yaml file. Each entity will have one or more components associated with it. Any entity defined in the yaml file needs to be scheduled by a scheduler. The sample makes use of GreedyScheduler. All schedulers are associated with a clock and RealtimeClock is chosen.
Graph Composer package provides tool to execute applications. The execute_graph.sh script located
at /opt/nvidia/graph-composer/execute_graph.sh
helps install the graph using registry and then executes it.
$ /opt/nvidia/graph-composer/execute_graph.sh --help
$ /opt/nvidia/graph-composer/execute_graph.sh -d /opt/nvidia/graph-composer/config/target_x86_64.yaml apps/app.yaml
This will print the following logs generated by the test component among others
Replace implementation in tick() and start() with custom implementation.